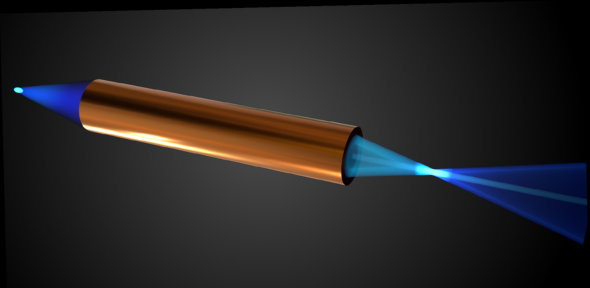
How can you deliver radiation in free space from one point to a distant focused spot? Recently, Cavendish researchers from the Semiconductor Physics group have reported a new method of solving this task using only cylindrical multimode waveguides.
The problem of delivering and focusing radiation from one point to another is common to any optical experiment. Usually it is solved with the help of two lenses: one that collimates the beam from the source, and another one that focuses the beam of parallel rays to a point. Another way of solving this problem is to use two parabolic mirrors. This is a special kind of mirrors, well known for their use as satellite dishes, that rely on reflection for focusing, instead of refraction, as lenses do. Scientists from the Semiconductor Physics group have now demonstrated an alternative way of accomplishing this task – using nothing more than a single cylindrical multimode waveguide.
We have asked them how it works, and why it matters.
What is a cylindrical multimode waveguide?
“Essentially, it is just a cylindrical metallic tube or pipe,” explains Prof. David Ritchie, Head of the Semiconductor Physics group at the Cavendish Laboratory. “We use it to guide radiation, so the tube becomes a waveguide, in physical terms. We do experiments in the terahertz range, which is the frequency region between the microwave and infrared ranges of the electromagnetic spectrum. The typical wavelengths are on the order of a fraction of a millimeter – around 0.1 to 0.3 millimeters. The cylindrical tube that we used has a diameter of about half a centimeter, which is much larger than the wavelength of the light. So, if we send terahertz radiation through it, this means that many modes, or patterns of radiation, can go through the waveguide at the same time. Therefore, it is a cylindrical multimode waveguide.”
How can you focus light using a cylindrical tube?
“We start with positioning the tube such that both the source of radiation and the point where we want the radiation to become focused lie on the axis of the cylindrical tube,” describes Dr Wladislaw Michailow, Junior Research Fellow of Trinity College and postdoc in the Semiconductor Physics group. “Then in this case, radiation emitted from the source will be reflected at the walls of the tube and redirected back onto the axis. You can compare this effect to lenses: A normal (convex) lens redirects parallel incident beams onto its focus using refraction. In our case, a cylindrical tube focuses radiation that hits the inner walls using reflection onto the tube’s axis.”
But this redirection of radiation would just result in a higher intensity on the waveguide axis, but not really in a proper focus, wouldn't it?
“Indeed, that is right,” confirms Wladislaw. “If you try to use this principle with an LED or a small lightbulb, that is exactly what you will see: an increased intensity on the waveguide axis. That is because this light is broadband in frequency and incoherent. What we have not considered so far, are interference phenomena. If you have coherent light with a single wavelength, which is generated by a laser for example, then individual reflections from the waveguide walls will interfere with each other, which leads to constructive and destructive interference. As a result, a beautiful pattern of intensity maxima and minima emerges within the waveguide and after its end. If you solve this optical problem mathematically, you can find conditions when all the reflected radiation interferes destructively everywhere except on the waveguide axis. This situation is achieved when the physical dimensions of the waveguide and the wavelength have a certain well-defined relation between each other. Then you get a nice focal spot located some distance away from the waveguide end, similar to what you can obtain with a pair of lenses.”
But why would you not ... simply use lenses?
“It would be nice if we could,” smiles David. “They are great workhorses in benchtop optical setups, but in fact, lenses have only limited applications. Specifically, in our research we aim to deliver terahertz radiation to low temperature environments in cryostats. Such cryostats have very small sample spaces. This makes it hard to insert any lens or mirror holders, and also to ensure that they do not compromise the cooling performance.”
“Thanks to this waveguide focusing effect, we made an experimental setup that combines four areas: electrical measurements, terahertz excitation, access for visible or near-infrared light, and all of this at liquid helium temperatures of –268°C,” adds Wladislaw.
What practical applications could this waveguide focusing effect have?
“The effect can have many applications in material characterisation,” explains David. “Terahertz spectroscopy is successfully used to study thin layers, for example to measure the thickness of material coatings in a non-damaging way. The waveguide focusing effect can help expand such technology to material characterisation inside of narrow, deep holes in components, or to study degradation inside of a car or aircraft engine without having to fully disassemble them.”
“Another application area is medicine,” says Wladislaw. “Terahertz spectroscopy allows to see skin cancer even in areas where you can’t see it with the naked eye. But terahertz photons have low energy and are easily absorbed in water. On the one hand, it makes them harmless compared to x-rays, but on the other hand, it means that you can't see through the body tissue with them. So at the moment, such techniques can only practically be used on the surface, i.e. the skin. But with the waveguide focusing effect, it would be possible to use a long, narrow terahertz probe e.g. during a chirurgic operation to obtain precise information about diseased tissue in previously inaccessible areas. Trying to fit in a whole setup with lenses or parabolic mirrors would be a bit problematic. Thus, systems with narrow waveguide tubes exploiting the focusing effect can open up new opportunities in these areas.”
This work was supported by Trinity College Cambridge and the EPSRC programme grants HyperTerahertz (No. EP/P021859/1) and TeraCom (No. EP/W028921/1).
Reference:
Wladislaw Michailow, Nikita W. Almond, Harvey E. Beere, and David A. Ritchie, 'Cylindrical Multimode Waveguides as Focusing Interferometric Systems', ACS Photonics (2023), DOI: 10.1021/acsphotonics.2c02030
Images:
1 : Cylindrical multimode waveguide as a focusing optical element (A 3D illustration of how the focusing effect looks like.)
2: Radiation propagation through a cylindrical multimode waveguide in cross-section
Credit: Wladislaw Michailow